Evaluation of a weathered rock aquifer
Abstract:
In areas where weathering has hydrogeological significance, geophysical methods can
assist to map the subsurface characteristics for groundwater occurrence. In this study, electrical
resistivity tomography (ERT) survey in combination with joint profile method (JPM), magnetic
method and borehole data was conducted to investigate the aquifer potential in strongly weathered
volcanic rocks. The aim was to assess the geological units related to the water-bearing formation of
aquifer systems in South Guangdong, China. The resistivities were measured along four profiles
each with a total of 81 electrodes, a spread length of 400 m and an electrode spacing of 5 m insuring
continuous coverage. The data from a borehole survey revealed three different layers i.e., highly
weathered layer, partly weathered layer and fresh basement rock, whose respective thickness were
integrated into ERT images to get more useful results about the real resistivity ranges of the these
layers (i.e., 22 Ωm–345 Ωm for highly weathered layer, 324 Ωm–926 Ωm for partly weathered
and 913 Ωm–2579 Ωm for fresh bedrock). The electrical resistivity imaging including the surface
topography provides spatial variations in electrical properties of the weathered/unweathered layers
since resistivity depends on the properties of a material rather than its thickness. ERT sections were
integrated with JPM and magnetic method to delineate the main faults (F1, F2 and F3). ERT sections
show a geometric relationship between different layered boundaries, particularly those of the aquifers
with fresh basement and surface topographies. These layers comprise an overburden of 50 m thickness
revealed by ERT sections. The results show that weathered and partly weathered layers between the
topographic surface and bed rock yield maximum aquifer potential in the study area. ERT imaging
method provides promising input to groundwater evaluation in the areas of weathered environment
with complex geology
Keywords:
Electrical Resistivity Tomography (ERT); pole-dipole configuration; groundwater;
geophysical method; weathered rock; Guangdong
1. Introduction
Electrical resistivity tomography (ERT) is an effective tool to investigate the hydrogeological
characteristics of the subsurface materials. When using this technique, the target is not only the
groundwater itself but also the geological structures and materials that are capable of storing and
transmitting the groundwater. Since the 1990s, the applications of this method have increased due to the
advancement in data acquisition techniques, interpretation techniques and computer technology [1–5].
As a result, it has become more precise and efficient to map the complex and small-scale geological
features [6,7]. The electrical methods can assist to assess the subsurface in areas where tectonics and
weathering have hydrogeological significance. Several studies have been reported over conductive
Water 2018, 10, 293; doi:10.3390/w10030293 www.mdpi.com/journal/water
Water 2018, 10, 293 2 of 22
and resistive intrusive bodies using resistivity soundings and profiling surveys [8–10]. Resistivity is
a measure of the resistance to electrical conduction for a material [11]. It is a property of the material
which depends on its lithology, water content, porosity, and temperature etc. but not on its size and
shape [3,11,12]. The main effect is for pore fluid (usually water content) except for conductive minerals
such as clay, sulfides, graphite etc. [11,12]. It decreases with increasing clay content [4,11]. It increases
with temperature for most of the materials except semiconductors (e.g., silicon) [11]. It rapidly
decreases with the water content [3,4,11]. Generally, resistivity of igneous/metamorphic rocks is the
highest followed by sedimentary rocks and soils [13–15]. Furthermore, computer-based resistivity
surveying has been employed over fractured, fissured, and weathered rocks [16,17]. Geophysical
methods have been very successful to map the subsurface for groundwater occurrence [18,19].
The electrical methods can be used to explore the groundwater conditions where the borehole
information is scarce [20]. These methods can be successfully applied to characterize the weathered rock
areas, as distinctive contrast is clearly observed on reaching the fresh bedrock [21]. Many researchers
have examined the weathering response to the electrical properties including the relationship between
weathered rocks and high resistivity responses [22–26]. The use of 2D resistivity imaging method has
been suggested because of the lateral resistivity heterogeneity generally associated to the weathered
rocks [27]. Diverse data collection geometries exist for 2D resistivity imaging and must be chosen
depending on the aims. Self potential (SP) surveys have been used to assess the subsurface conditions
for groundwater flow [28–30]. Resistivity and induced polarization (IP) are the commonly used
geophysical methods to map the electrical properties of the subsurface rock [31,32]. The Wenner,
Schlumberger, pole-pole, dipole-dipole, and pole-dipole are the most commonly used arrays. The latter
array for the differentiation of the complex geological structures has been used affectively in many
studies [33–35]. The pole-dipole array was used in the present study because it has significantly higher
signal strength to get high resolution of 2D ERT data and is less affected by the remote electrode, and
it has more vertical sensitivity and large depth of investigation [11,36,37]. Recently, the applicability
of the multiple gradient arrays for multi electrode surveys has been affectively used [38]. In the
mountainous areas, the geo electric surveys can be affected by the lateral surface irregularities and high
slopes regardless of the used array. The topographic changes produce the distortions in the measured
potential field that can cause the terrain anomalies [39,40]. These topographic variations are accounted
by the integration of altitude data in a finite element grid for the inversion.
The objective of this study was to assess the hydrogeological materials and the structures of
the formations for groundwater occurrence along four profiles in the topographic Huidong area,
South Guangdong, China. In this study, ERT imaging method was used to map the distinct subsurface
layers in the weathered volcanic rocks for the evaluation of groundwater potential. This study
highlights the effectiveness of the ERT resistivity imaging method to map the potential subsurface
layers for aquifer occurrence in the weathered environment as well as the importance of using the
topography in the surveyed areas where the topography changes dramatically. This 2D resistivity study
was carried out with four profiles each yielding 81 electrodes, 5 m electrode spacing and a spread length
of 400 m at hydrogeologically relevant locations of Huidong area in South Guangdong province of
China (Figure 1). ERT sections were integrated by JPM and magnetic method to identify the main faults
along all profiles by observing the alterations in resistivity, self potential (SP), induced polarization (IP)
and magnetic curves. Four profile lines of resistivity measurements are employed in the area so that
they can reveal the typical responses from the geological materials and structures for groundwater
investigation in the study area. To reinforce the field data interpretation, the pole-dipole electrode
arrays along four different profiles were tested to correlate with the synthetic models of geological
strata expected to occur in the study area. The investigations were carried out within the framework of
a multi-disciplinary research supported by Institute of Geology and Geophysics, Chinese Academy of
Sciences with the aim to map the groundwater systems in the area of weathered environment.
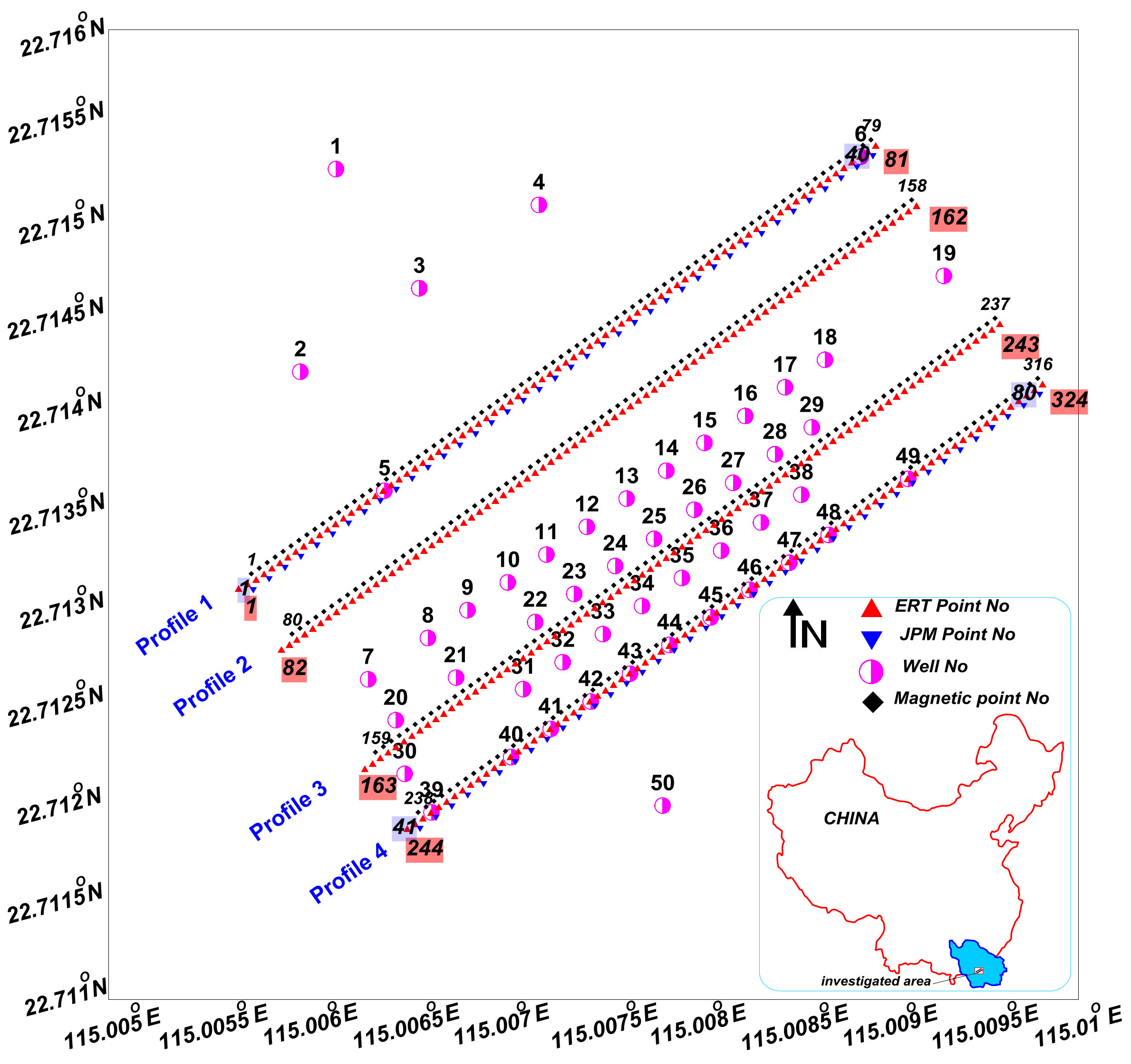
2. Study Area and Hydrogeological Setting
Huidong is in the southeast of Guangdong province, China with an area of 3397 km2
. It lies in the
longitude from 114.3◦ E to 115.3◦ E and the latitude from 22.3◦ N to 23.2◦ N. The study area is situated
in the tropical monsoon climate of South Asia with an annual rainfall of 1859 mm. Monsoon is the
rainy season in the investigated area starting from April to October, and the typhoons come from June
to October. It has short winter and long summer with mean temperature of 21.7 ◦C. The maximum
temperature in summer is 40 ◦C, and minimum temperature in winter is 5 ◦C. Huidong can be divided
into three main parts based on geomorphology, i.e., the eastern mountains, the middle hilly area,
and the south hilly area along the South China Sea.
The simplified geological settings of the study area are given in the hydrogeological map as
shown in Figure 2. Based on the geological settings, the investigated area is composed of volcanic and
magmatic rocks/minerals of Jurassic age such as volcano dust, matrix, quartz, pyrite sulfide, feldspar,
pyroxene, and basalts with some quartz veins embedded in the Aeolian tuff rock [41]. Various factors
control the groundwater potential, occurrence and its flow in the study area such as hydrothermal
processes, tectonic forces associated with weathering, fracturing, and fissuring, and secondary
alteration of veins that contains high concentration of structural, lithological, and compositional
heterogeneities that cause the water circulation [41]. The weathering processes play a major role
to facilitate the water infiltration and its transportation in the top surface layer. Hydrogeologically,
the weathering assists to make up the crucial period for the occurrence of groundwater reserves [42].
In the investigated area, the main aquifer is found in the upper layer formed by weathering, fissuring,
and fracturing. Several boreholes were conducted in this investigation to reveal the existence of
weathering, fissuring, and fracturing in the subsurface formation. Generally, the water level varies
from 2 m to 32 m below the top surface, and from 106 m to 148 m with the elevation. In addition
to above factors, various faults, the dynamo metamorphic zone and an unconformable boundary
make the hydrogeological conditions more complex in the area to study the groundwater potential
in hard rock aquifer (Figure 2). The topographic variations in the study area are shown in Figure 3.
Electrical resistivity tomography (ERT) survey integrated by borehole, JPM and magnetic method was
conducted in Huidong area by a joint project of University of Chinese Academy of Sciences, Institute of
Geology and Geophysics to assess the subsurface hydrogeological conditions for groundwater potential.
Figure 1 shows the location of the study area with the measurements of ERT, JPM, magnetic and borehole data.
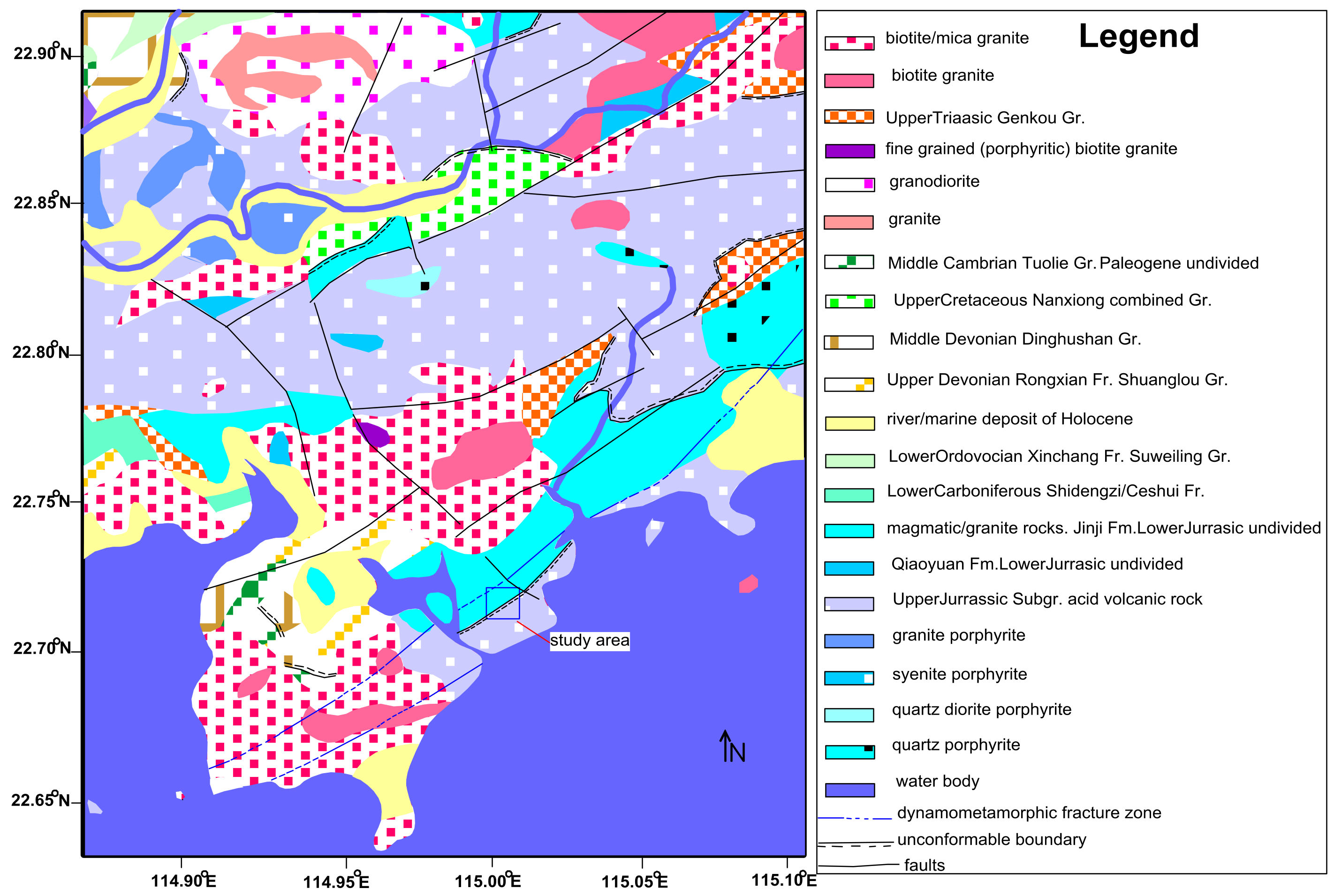
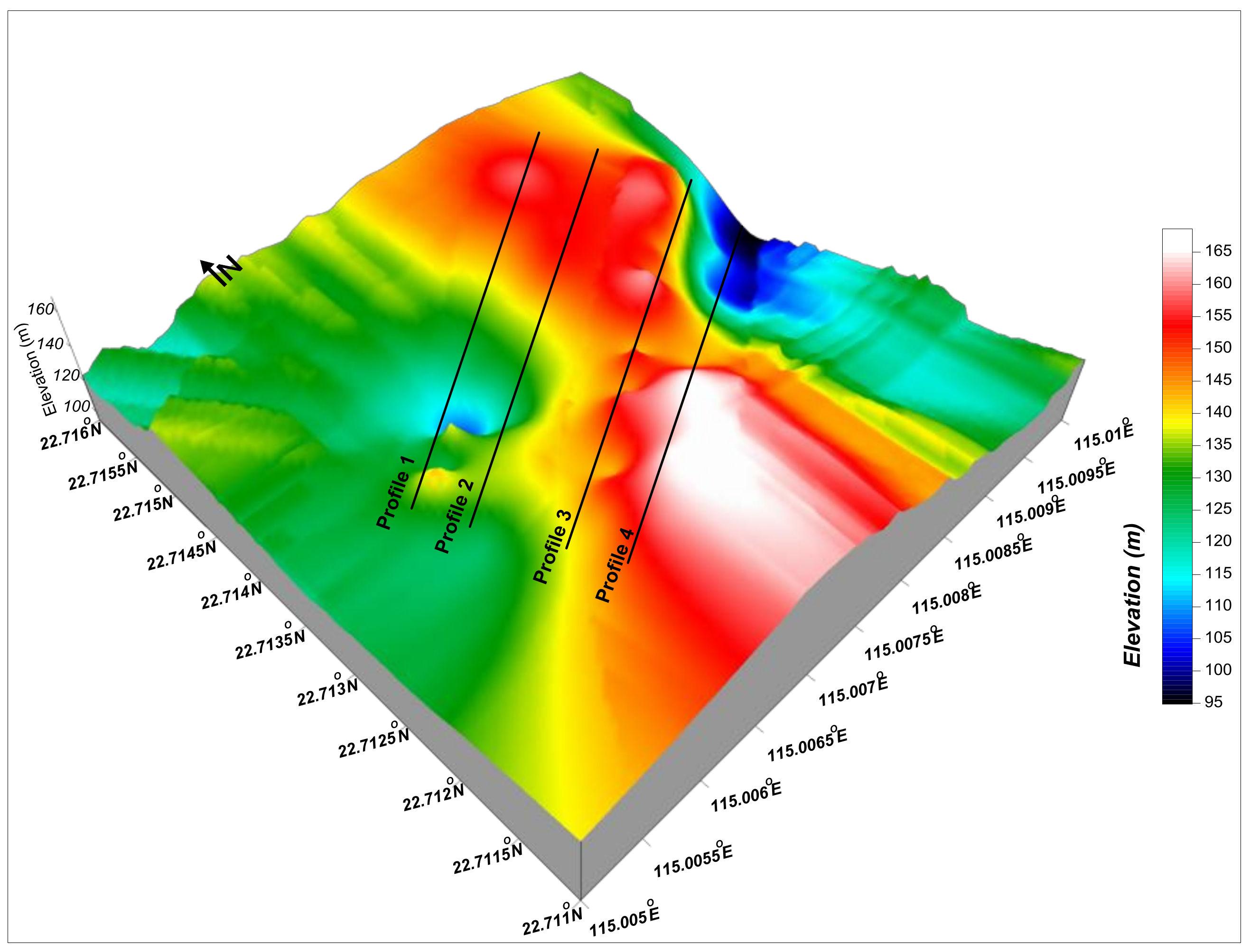
3. Materials and Methods
In this survey, ERT method with ABEM Lund Imaging System (Lund University, Lund, Sweden) [16]
was applied using different layouts. This is an automated imaging system for the collection,
processing, and presentation of resistivity. This method is based on the conductivity difference
between the rock formation and the surrounding materials. By studying the underground steady
electric field, this method is a very useful exploration tool to solve the engineering hydrogeological
problems. The resistivity imaging is a recently developed modern technique to investigate the
subsurface characteristics of the areas with complex geological settings where the applications of
other methods such as resistivity soundings are not affective [43]. This method is defined by the
density, the arrangement of electrodes and the amount of information to be acquired. ERT provides
the subsurface geologic information through the corresponding interpretation using a software.
Water 2018, 10, 293 5 of 22
An example for the principle of building up a resistivity pseudo-section using ABEM Terrameter
with a system of 20 electrodes for pole-dipole array is given in Figure 4. It is a multi-function electrical
instrument that has a multi-electrode convertor for the observations. Electrical resistivity tomography
(ERT) is cost effective, fast, and versatile technique for mapping the groundwater conditions and
assessing the shallow subsurface anomalies [44]. This technique is useful to obtain the important
information for the planning of groundwater exploration. By increasing the electrode spacing, it can
measure a series of constant separation traverses. Since increasing the separation provides more
depth penetration, the vertical contoured image of measured apparent resistivity shows lateral and
vertical variations in apparent resistivity over the section. The important geological units such as
weathered/partly weathered/fissured layers, fractures and faults associated with the groundwater
occurrence in the area were the main targets under this investigation. Different rock types (weathered
rocks, partly weathered rocks, unweathered rocks), fractures, fissures, streams, or springs in the
surroundings were considered as important aspects in selecting the locations for the resistivity
lines. It was also very important to get the useful geological information in situ by drilling the
boreholes. The slurry hole-boring method was used for drilling without using any metallic casing in
this investigation.
Electrical resistivity tomography (ERT) is a complete automated technique that is employed using
several electrodes joined by a multicore cable. The positions of current and potential electrodes are
adjusted by a resistivity meter using a microprocessor controlled electronic module. Before starting
the field measurements, a specific electrode configuration with fixed electrode spacing and all other
required information is provided to the resistivity meter through a computer and resistivity data is
collected automatically through this arrangement. To get the accurate position of the profiles and
electrodes, surveying instrumentation and GPS systems (MAP60, Garmin, Olathe, KS, USA) were
used in the field measurements. Commercially available software (prosys-geotomo software) is used
to process this field data in different stages. The obtained apparent resistivity data is inverted by
the software to generate a 2D image of the modeled resistivity along the profile. 2D as well as a 3D
image of the subsurface can be obtained using the proper survey pattern and data procedure. In this
study, 2D resistivity imaging was used along four profiles. Pole-dipole configuration was used to
collect the resistivity data along four profiles with remote pole at a distance of 3 to 4 times the array
length, and with each profile carrying a total of 81 electrodes, 5 m electrode spacing and a spread
length of 400 m. The data acquisition was performed for 25 layers along each profile. The data were
collected by applying a Terrameter SAS4000, ES10-64C switcher (Lund University, Lund, Sweden) and
12 V battery. The topography along the profiles was obtained with the measurements of every 10 m
using a clinometer. The signal to noise ratio was improved using a single point data collected with
maximum 10 stacking. During the processing of resistivity data, the bad quality data were filtered,
and good quality data were used as input to RES2DINV software [3,12,45,46]. RES2DINV software
(v3.50, Geotomo software Company, Penang, Malaysia) is an inverse program that is used to generate
a 2D resistivity picture of the subsurface. RES2DINV is a least-squares inversion technique that must
apply a smoothness constraint [47,48]. This inversion procedure generates a smooth model that can
fit the data to a given level of error. In this survey, RMS error (difference in calculated and apparent
resistivity values) for all inversion models was less than 5%. This model consists of several rectangular
blocks equal to the data points of the resistivity pseudo-section. The central depth of the interior blocks
is placed at the median depth of investigation [49]. The conventional Gauss-Newton least squares
method [50] is an inversion scheme that can be used to minimize the difference between measured
and modeled resistivity data. The smoothness-constrained modified model of Gauss-Newton [47] is
based on the following equation:
(Ji T Ji + λiC TC)pi = Ji Tgi
where
i = iteration number
Water 2018, 10, 293 6 of 22
Ji = Jacobian matrix of partial derivatives,
gi = discrepancy vector
λi = damping factor
pi = perturbation vector to the model parameters for the ith iteration
C = 2D flatness filter
where gi depends on the difference between the logarithms of the measured and calculated values of apparent resistivity, C is applied for constraining the smoothness of the perturbations for some constant value of the model parameters [51], and λ depends on the random noise of the data and its large value is used for high noise. The partial derivatives of a block are independent of the block size, which decrease with increasing distance to the electrodes. The amplitude of C for each row increases by 10% for stabilizing the inversion. C TC is sparse and symmetric. The first step in the least squares inversion is to calculate the apparent resistivity, the second step is to calculate Jacobian matrix J and the third step is to solve the above system of the linear equations. The finite difference or finite element methods can be used to complete the first two steps, whereas the third step can be solved using number of techniques such as the modified Gram-Schmidt, Cholesky and singular value decomposition methods [52].
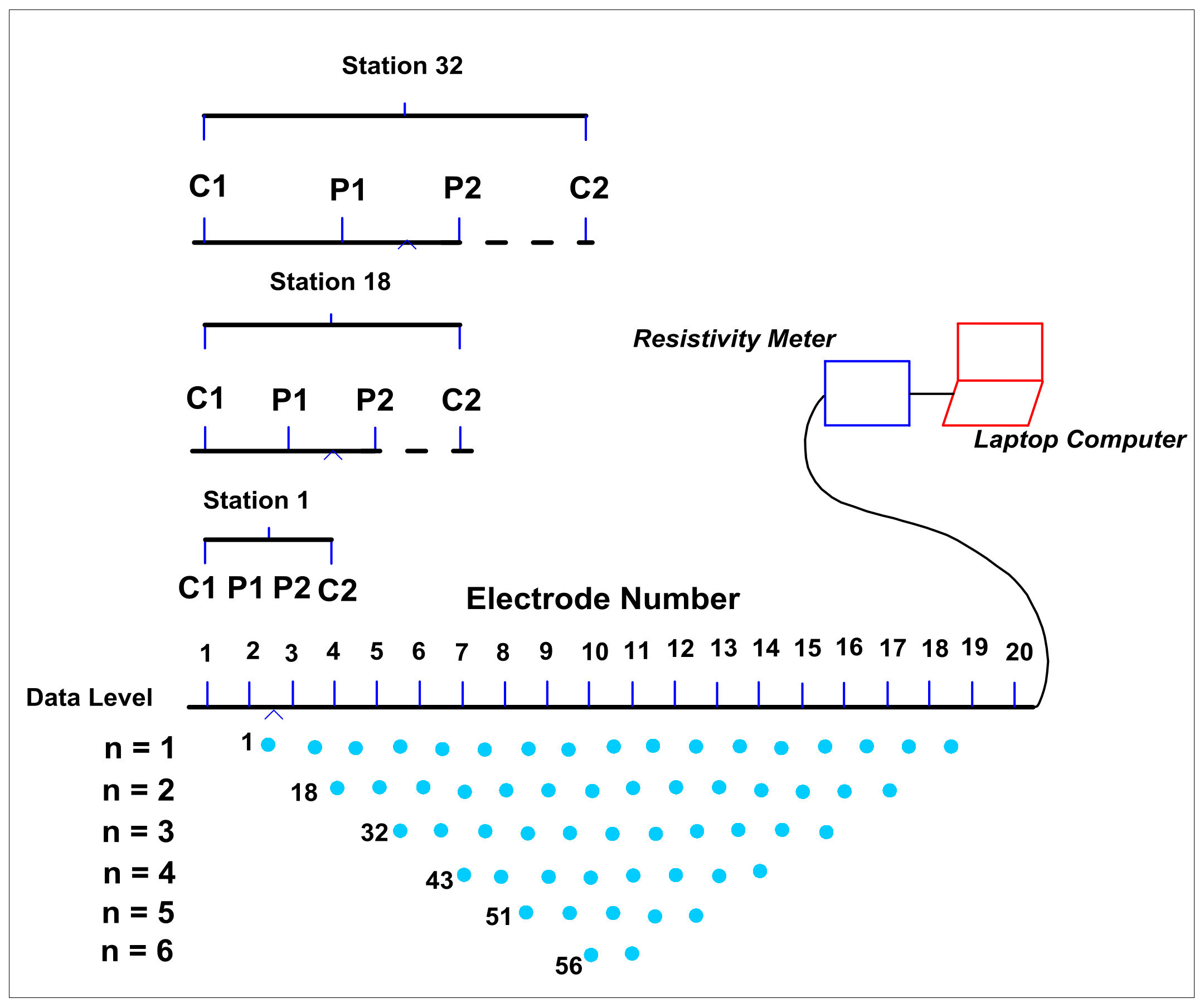
In this study, ERT provides the subsurface image up to 50 m depth. The depth of the investigation depends on the factors such as array type, number of electrodes, electrode spacing, number of segments and the ability of equipment to measure the signal (i.e., amplitudes of the signal and the noise, power specification of the equipment and its ability to filter the noise through stacking process). To calculate the maximum depth of the investigation, the maximum electrode spacing or the maximum array length was multiplied by the ratio of median depth of investigation to the electrode spacing or the total electrode spacing [11]. To delineate the main faults along all profiles, ERT sections were integrated with Joint Profile Method (JPM) and magnetic data. Joint Profile Method (JPM) was performed along two of the four profiles to observe the alterations in resistivity, self potential (SP) and induced polarization (IP) at 90 m and 180 m depth (AB/2 = 90 m, MN/2 = 10 m and AB/2 = 180 m, MN/2 = 10 m, where AB/2 is the depth and MN/2 is the electrode spacing). JPM provides composite joint curves for resistivity, SP, and IP at greater depths (90 m and 180 m). In this method, a multi-function electric instrument W-3 Series (Chongqing Pentium Research Center, Chongqing, China) was used. The joint cross section device of JPM is shown in Figure 5. The joint curves of resistivity, SP, and IP show clear alterations along the main faults at greater depths which are consistent with the ERT sections. Magnetic data was Water 2018, 10, 293 7 of 22 plotted to observe the alterations in magnetic curves along the main faults, which shows consistency with ERT and JPM for the identification of main faults.
The topography and the lithostratigraphic information from the borehole data were integrated into the ERT profiles. After the data processing was completed, the results were interpreted for presentation by setting a resistivity range suitable for the expected hydrogeological features for each profile in the investigated area. A conceptual model of hydrogeological properties in the weathered environment of the study area was generated as shown in Figure 6. This model explains a complete concept for the groundwater occurrence in such complex weathered environment.
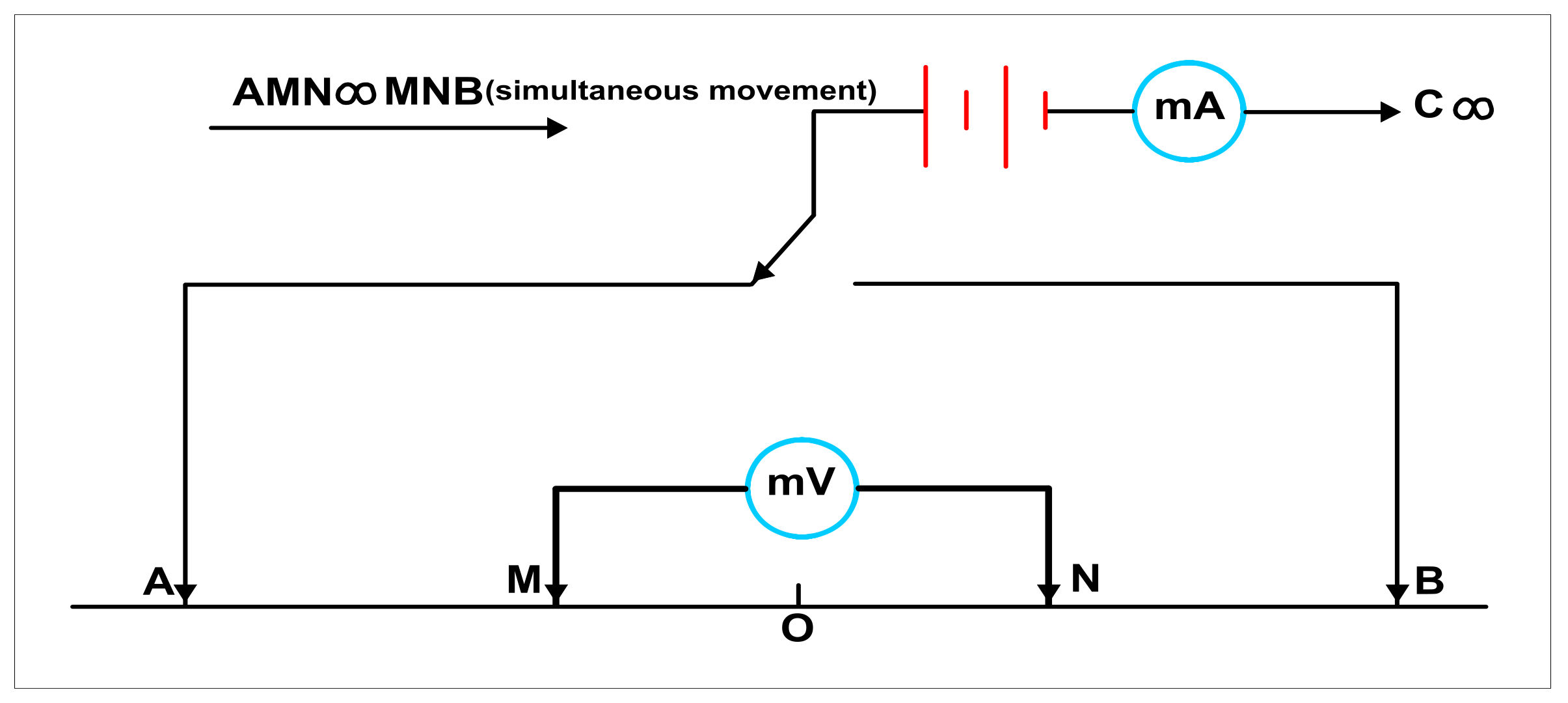
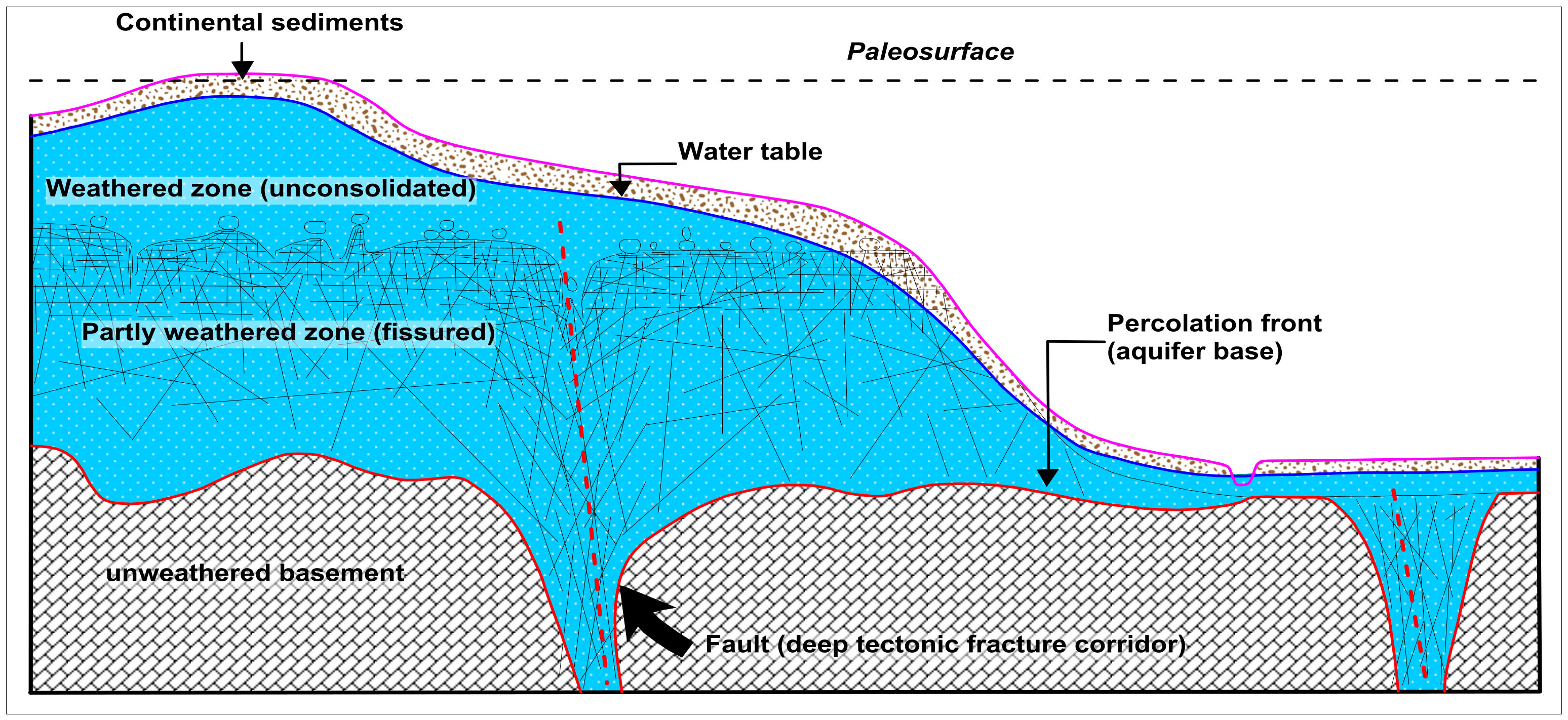
4. Results
Using the ERT method, the resistivity data were obtained and processed by RES2DINV software [3,12].
RES2DINV is a Windows-based computer program that is used to obtain a two-dimensional (2D)
resistivity image of the subsurface. The processed data show the modeled resistivity sections with the
topography along four profiles with the color infill. The data were inverted to obtain the ERT sections.
After the correlation of resistivity images with the borehole lithology, ERT sections were interpreted
into three layers with different resistivity ranges as shown in Table 1. The first distinct layer in the
model is highly weathered rock underlying the top surface with resistivity range of 22 Ωm–345 Ωm
and an average thickness of 20 m. Partly weathered rock is the second layer revealed underneath
the top layer with an average thickness of 10 m and resistivity ranging from 324 Ωm to 926 Ωm.
The fresh basement is the third layer encountered at an average depth of 20 m, its resistivity values
range from 913 Ωm to 2579 Ωm. Table 1 shows the main distinct layers revealed in the study area with
different resistivity ranges based on the calibration of resulted ERT sections with the borehole data.
Since the resistivity of the materials is inversely proportional to the percent water saturation, the low
resistive materials clearly show the water saturation. Furthermore, clay can also have a significant
effect on resistivity values. Resistivity decreases with the increase of clay content. Borehole data
Water 2018, 10, 293 8 of 22
revealed 2 m–5 m thick topsoil layer with silt/clay content in the study area. Figure 7 shows the
position of the water table in the study area. Generally, the water level in the study area lies within
the weathered rock; it varies from 2 m to 32 m with respect to the depth and from 106 m to 148 m
with respect to the elevation. The modeled ERT sections and supplemented SP and IP measurements
are shown in Figures 8–13. The modeled ERT sections are shown in Figure 8, and Figures 10–12.
Three main faults (F1, F2 and F3) were identified by the integration of ERT sections with JPM and
magnetic data. The delineation of the faults and weathered layers is very important because the
groundwater reserves are contained in the weathered/fault zones. The magnetic susceptibility of
weathered/fractured or fault zones is low, whereas it is high for unweathered/fresh basement rock.
Therefore, this significant susceptibility contrast between weathered/fault zone and unweathered
zone reveals a useful information for the groundwater occurrence in weathered/fault zone. SP and IP
measurements were used to supplement the ERT results for the delineation of main faults which contain
the groundwater reserves. SP is sensitive to the groundwater flow and its negative values suggest the
groundwater saturated fractured/fault zone. IP identifies the electrical chargeability of the subsurface
materials. A careful observation shows that faults zones containing the groundwater reserves in the
study area are characterized by low values of resistivity, SP and IP as shown in Figures 9 and 13.
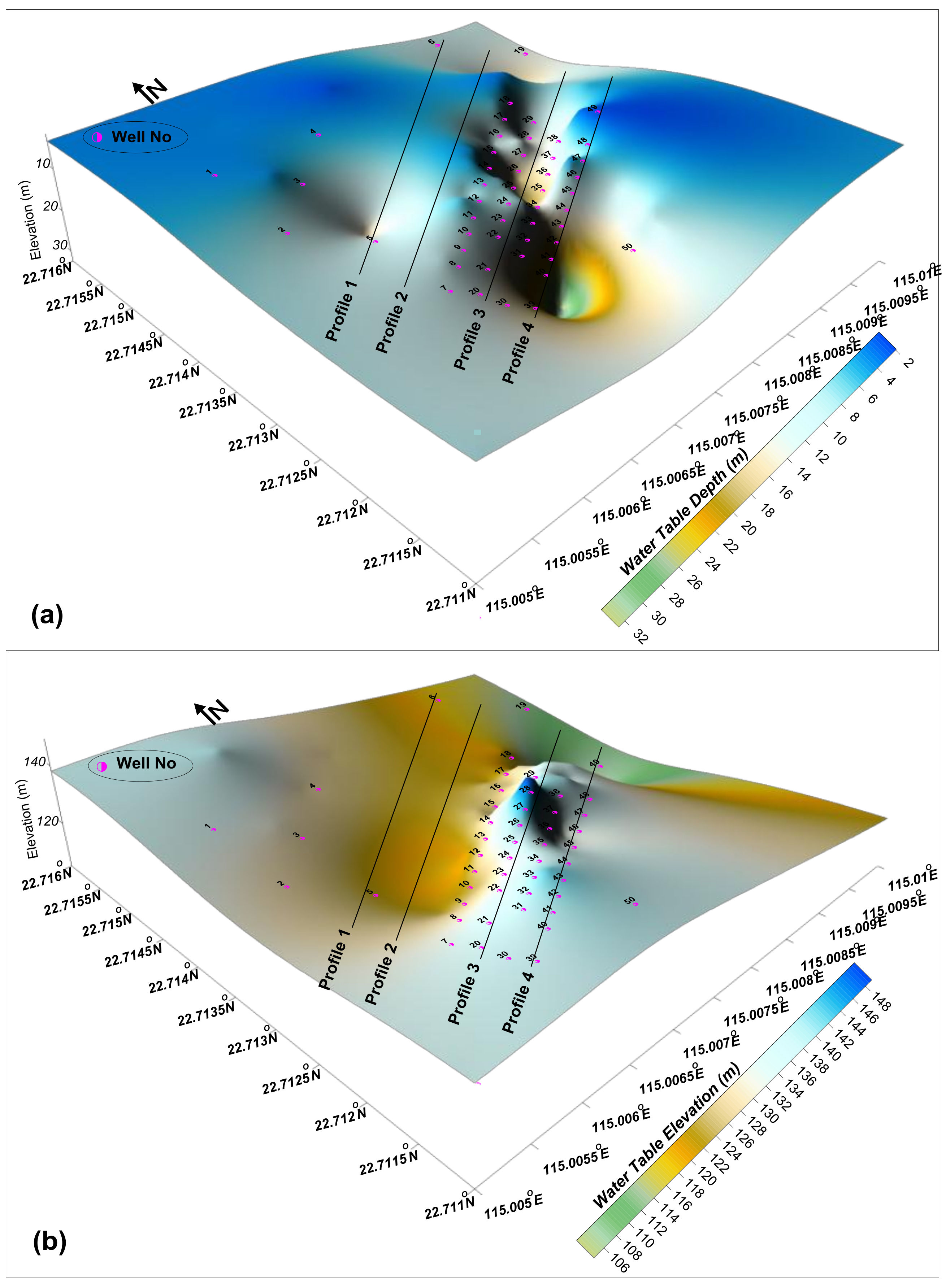
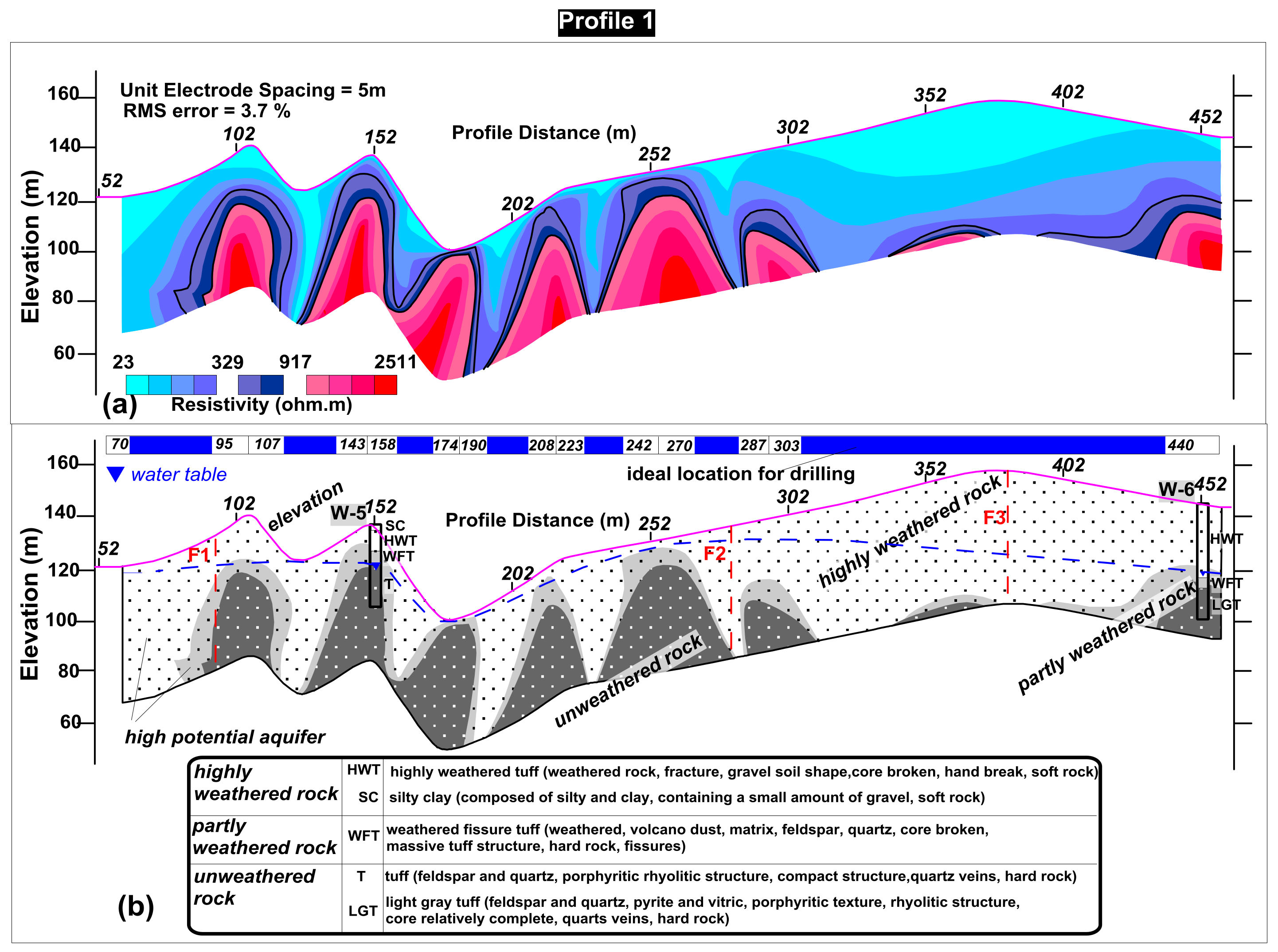
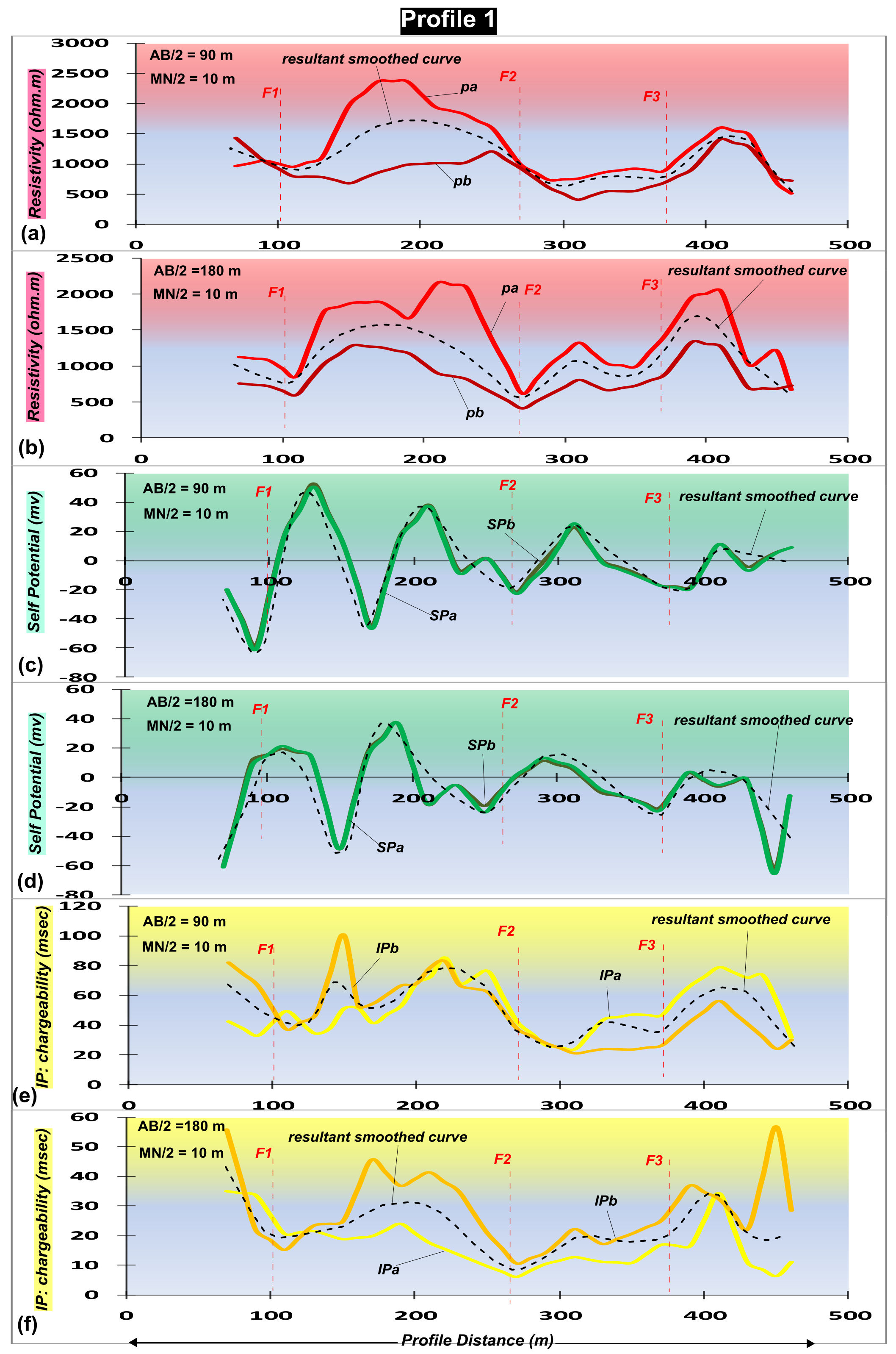
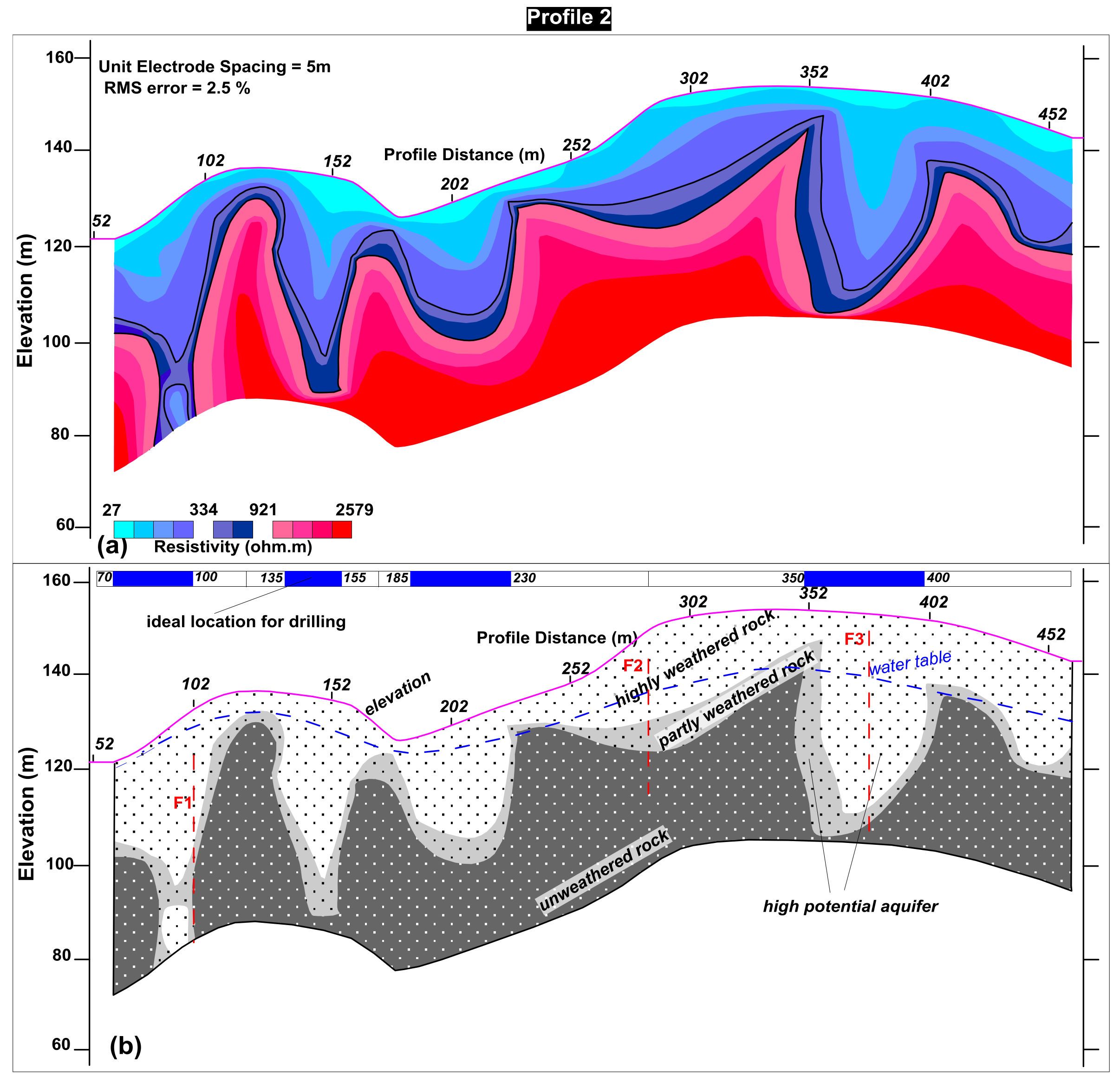
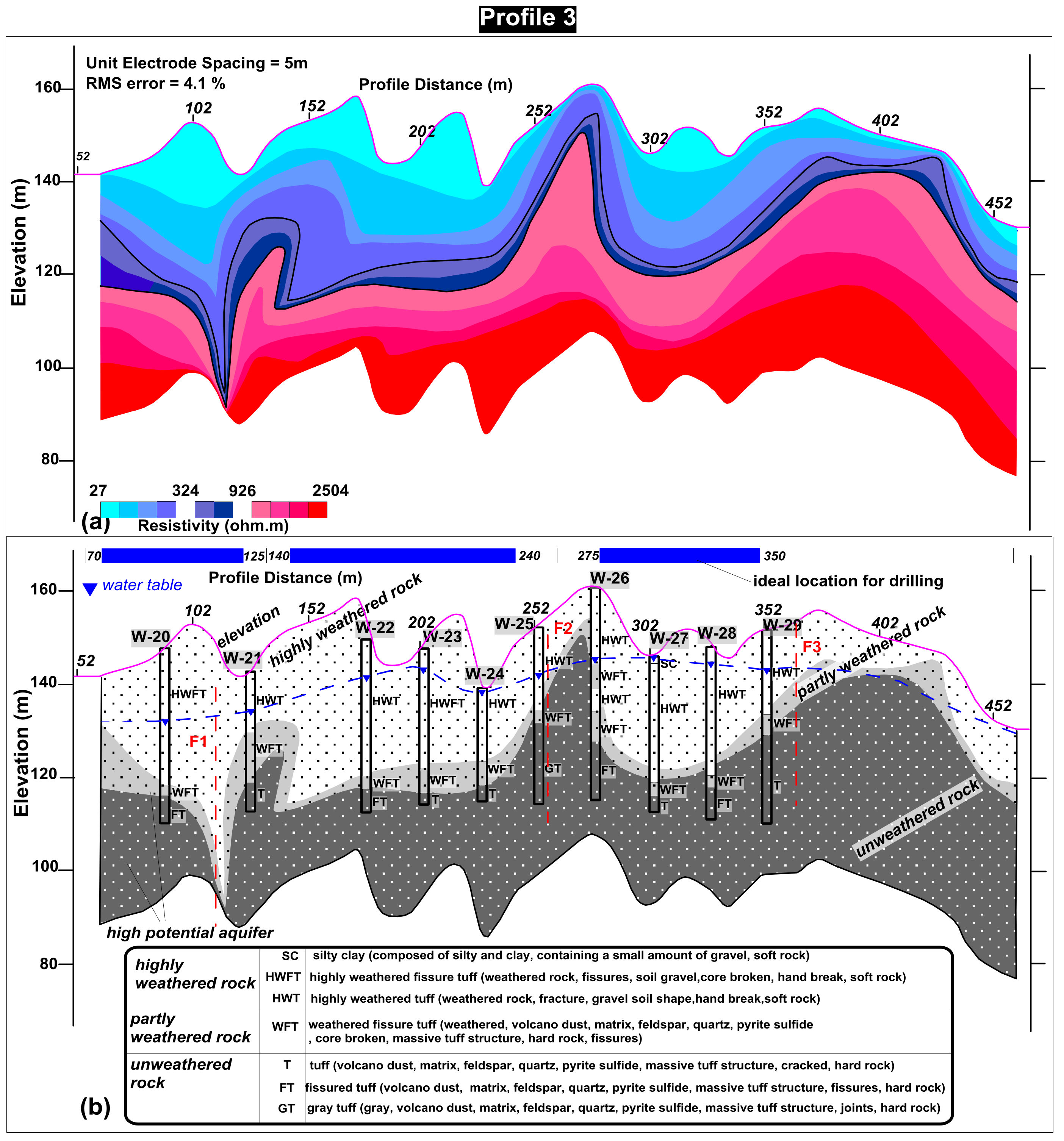
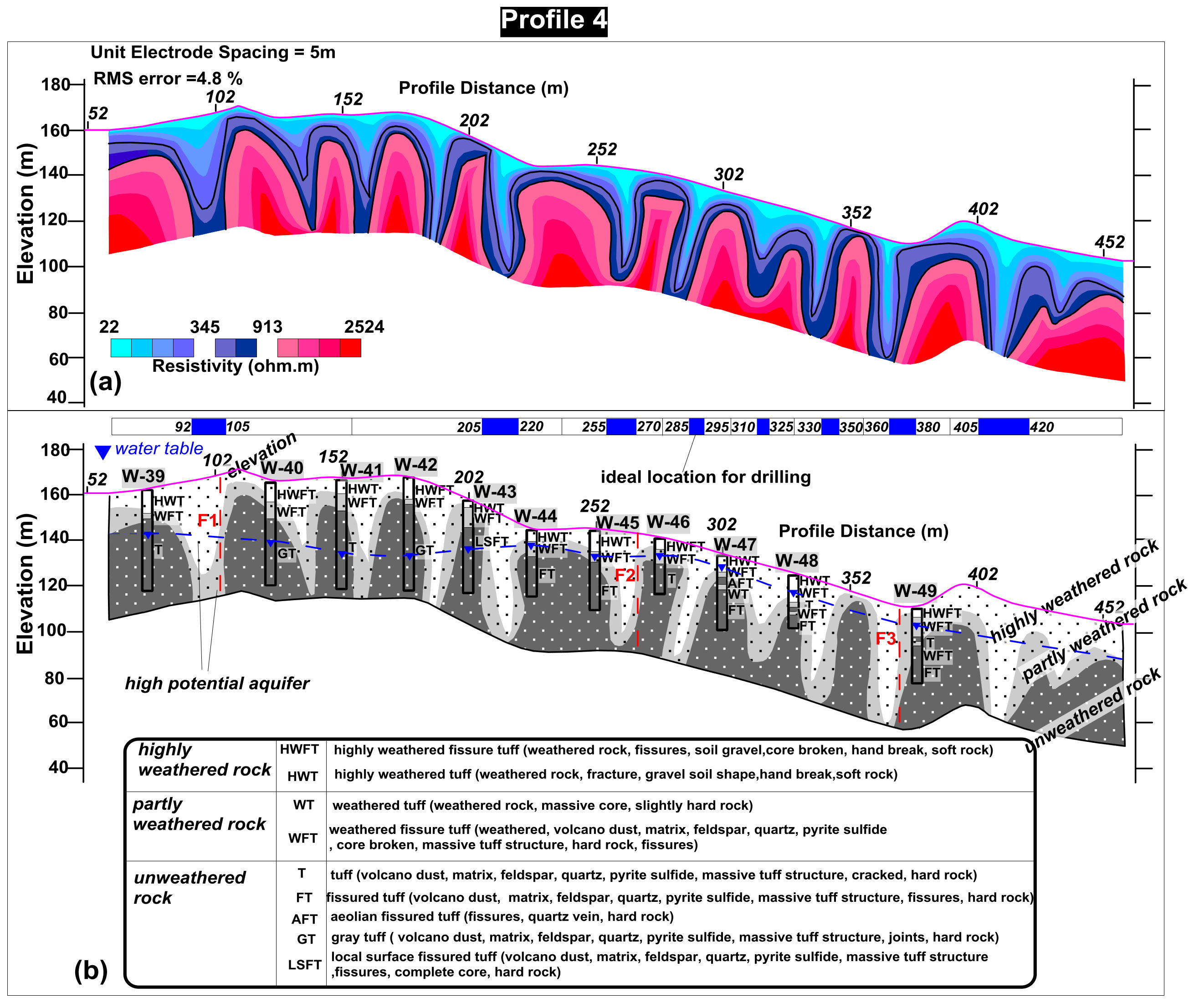
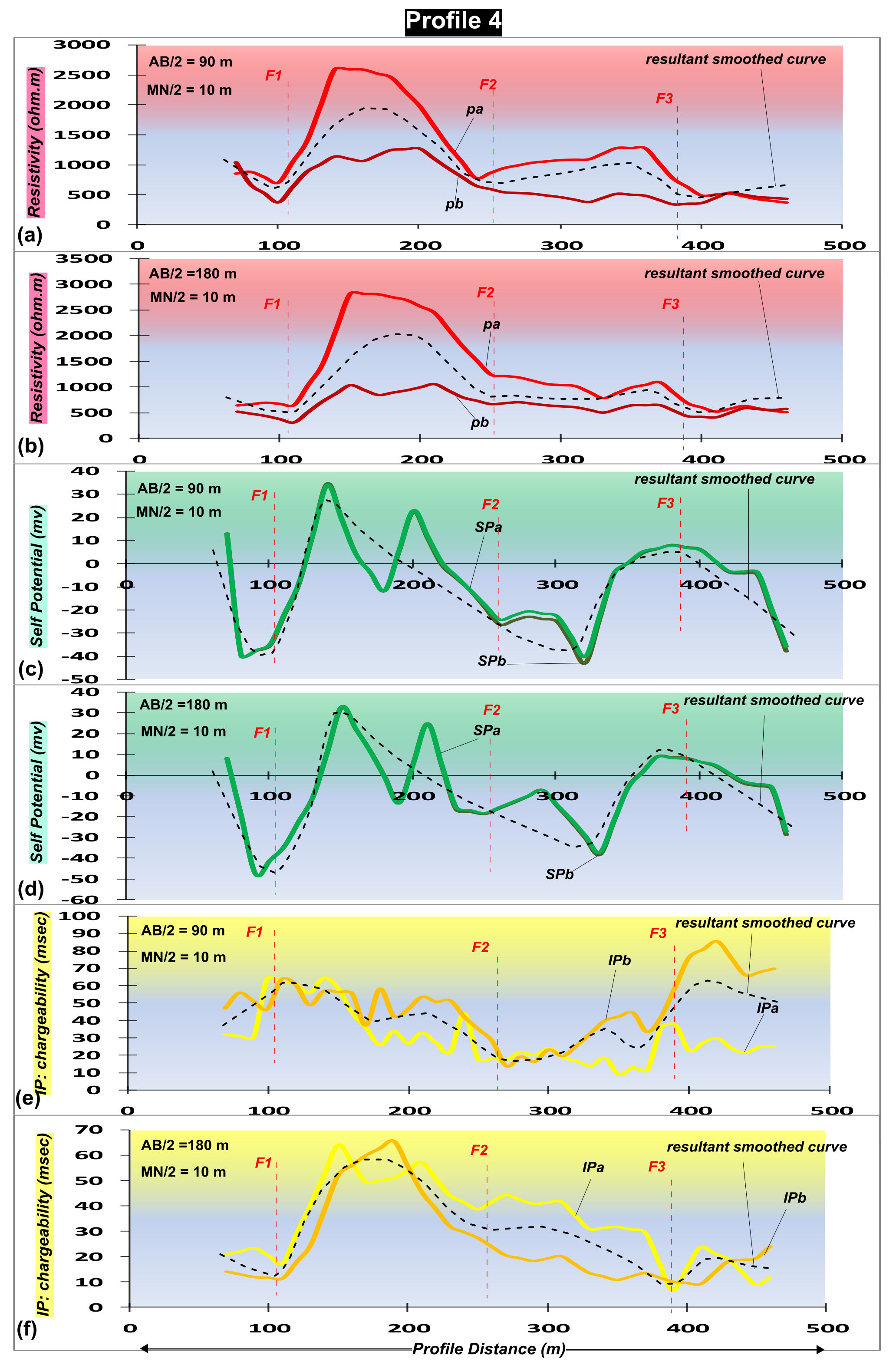
Table 1. Resistivity and lithology calibration in the study area.
4.1. Profile 1
The imaging method encountered three distinct layers namely highly weathered rock, partly
weathered rock, and the fresh bedrock along the profile 1 as shown in Figure 8. The top distinct layer
is highly weathered layer with an average thickness of 20 m, and it contains weathered tuff rock, silt
and clay and other weathered materials with resistivity ranging from 23 Ωm to 329 Ωm. The top layer
extends to the partly weathered layer which is 10 m thick and its resistivity ranges from 329 Ωm to
917 Ωm mainly composed of weathered fissure tuff rock with volcano dust, matrix, feldspar, quartz
etc. Fresh basement rock is encountered under the partly weathered layer at an average depth of
20 m with resistivity values ranging from 917 Ωm to 2511 Ωm. The bedrock contains unweathered
tuff rock mainly consisted of feldspar, quartz, pyrite etc. Groundwater in the Basement Complex
is generally found in the weathered, fractured, fissured, and jointed systems of the bedrock [55].
The low resistive layers indicate the presence of water. Highly weathered and partly weathered
layers are the most promising water-bearing layers in the study area. Figure 8 shows the interpreted
resistivity section of three distinct layers with the borehole information along profile 1. Three main
faults (F1, F2 and F3) were identified along this profile in northeast to southwest (South China Sea)
direction. The suitable locations for drilling were marked by the horizontal thick blue line as shown in
Figure 8b. The conditioning of groundwater is the thickness of the aquifer layer and Figure 8 shows
good locations to drill a borehole, for instance at 70 m to 95 m, 107 m to 143 m, 158 m to 174 m, 190 m
to 208 m, 223 m to 242 m, 270 m to 287 m and 303 m to 440 m.
Using JPM, the resistivity, SP and IP composite joint curves were plotted at 90 m and 180 m
depths to observe the alterations in the curves along the main faults as shown in Figure 9. Magnetic
data was also plotted to see the variations in magnetic values along the identified faults (Figure 14a).
The resistivity, SP, IP, and magnetic curves show clear alterations along the main faults integrated
by ERT.
4.2. Profile 2
No borehole was drilled along this profile 2 (Figure 10). Based on nearby borehole data, three layers
were identified (Figure 10). The first prominent layer under the top surface is highly weathered
rock mainly consisting of volcanic tuff rock and other weathered materials with resistivity range of
27 Ωm–334 Ωm and an average thickness of 20 m. The partly weathered bedrock is the next layer
underlying the top layer with an average thickness of 10 m and resistivity ranging from 334 Ωm to
921 Ωm. This layer is composed of weathered fissured tuff rock with volcano dust, matrix, feldspar,
quartz etc. Highly weathered and partly weathered layers are the best for groundwater occurrence
along this profile. The fresh bedrock is the third layer identified at an average depth of 20 m with
resistivity value as low as 921 Ωm and as high as 2579 Ωm mainly composed of unweathered tuff
with feldspar, quartz, pyrite etc. This layer is not ideal for aquifer occurrence, but it may be used as
pillar support for buildings or other engineering structures. The expected water level position along
this profile is also shown in Figure 10. Three main faults (F1, F2 and F3) were revealed by ERT and
magnetic data (Figure 14b) along profile 2 in the orientation of northeast to southwest. The ideal
location for drilling along this profile was marked from 70 m to 100 m, 135 m to 155 m, 185 m to 230 m
and 350 m to 450 m (Figure 10b).
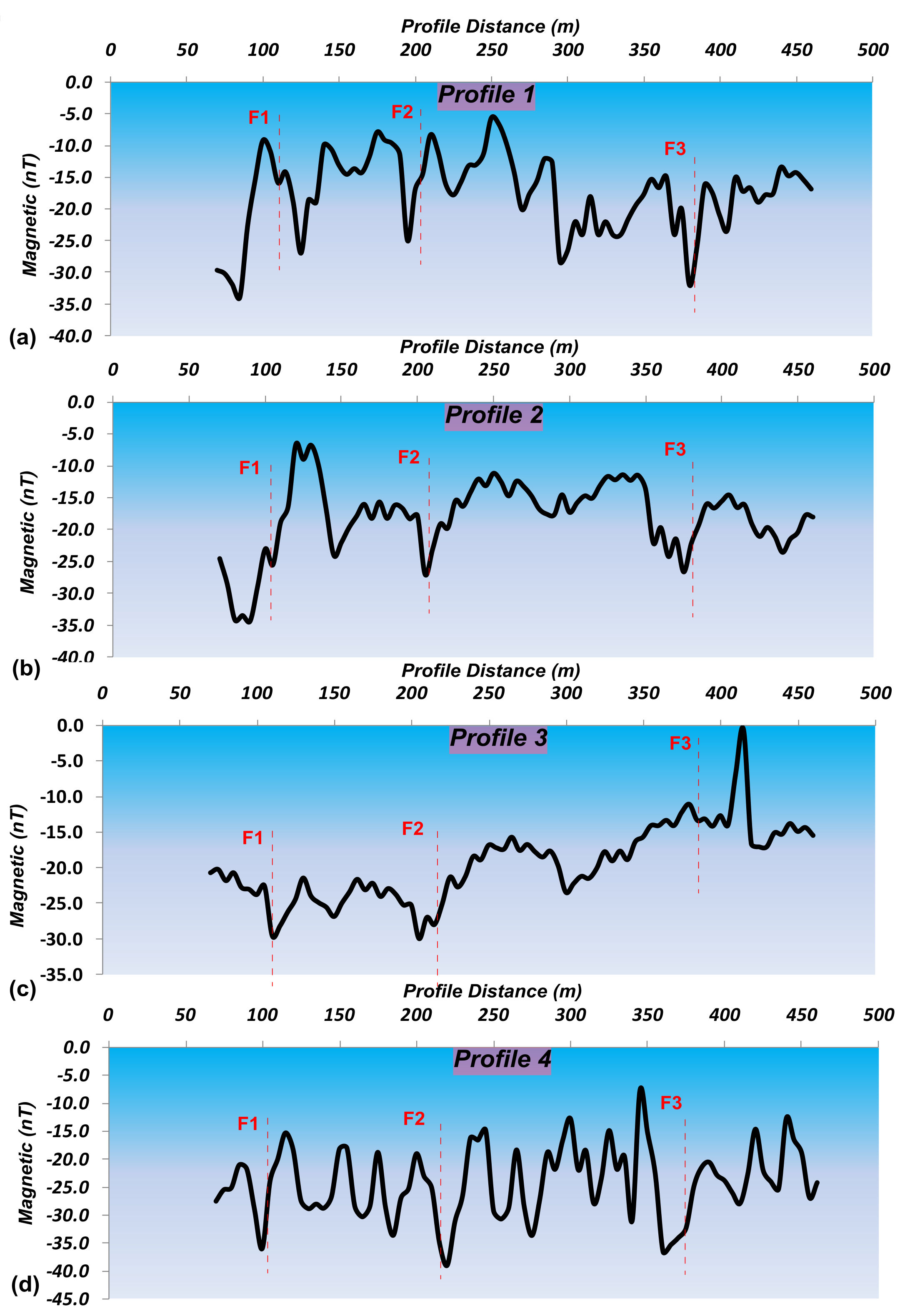
4.3. Profile 3
Ten boreholes were drilled along profile 3. This profile shows an electrical section of three layers
depending on the integrated results of ERT and the borehole data (Figure 11). The first distinct layer
exposed under the top surface is highly weathered, which has an average thickness of 20 m with
resistivity range of 27 Ωm–324 Ωm mainly containing weathered tuff and weathered fissure tuff with
silt and clay and other weathered materials. Underlying the top layer, a partly weathered layer is
revealed with resistivity ranging from 324 Ωm to 926 Ωm and an average thickness of 10 m. It consists
of weathered fissure tuff with volcano dust, matrix, feldspar, quartz, and pyrite sulfide. These two
layers are ideal location to drill the boreholes for groundwater exploration. At an average depth
of 20 m, unweathered layer is encountered which is the fresh basement rock with resistivity range
of 926 Ωm–2504 Ωm, it is mainly composed of tuff, fissured tuff and gray tuff containing volcano
dust, matrix, feldspar, quartz, pyrite sulfide etc. This layer is good for engineering structures rather
than aquifer potential. Figure 11 shows the position of water table which mostly lies within the
weathered layer. Three faults identified along first two profiles also extend to this profile with the same
orientation. The main faults (F1, F2 and F3) were delineated by the integration of ERT with magnetic
data (Figure 14c). The most suitable drilling locations along this profile were found at the distance
from 70 m to 125 m, 140 m to 240 m and 275 m to 350 m (Figure 11b).
4.4. Profile 4
This profile reveals three distinct layers namely highly weathered, partly weathered,
and unweathered layer, based on the lithology of eleven borehole data (Figure 12). The first layer
on ERT section of this profile is highly weathered mainly composed of weathered tuff, weathered
Water 2018, 10, 293 14 of 22
fissure tuff and other weathered materials with an average thickness of 20 m and resistivity range
of 22 Ω m–345 Ωm. Partly weathered layer is the next layer with resistivity ranging from 345 Ωm
to 913 Ωm and an average thickness of 10 m mainly consisting of weathered tuff and weathered
fissure tuff and other rocks/minerals such as volcano dust, matrix, feldspar, quartz, pyrite sulfide
etc. On reaching the average depth of 20 m, bed rock is encountered which is unweathered fresh
basement with resistivity ranging from 913 Ωm to 2524 Ωm mainly composed of fresh tuff, fissured
tuff, Aeolian fissured tuff, gray tuff, local surface fissured tuff with the rocks/minerals such as volcano
dust, matrix, feldspar, quartz, pyrite sulfide etc. Highly weathered and partly weathered layers are
the most promising water-bearing rocks along this profile. Three main faults (F1, F2 and F3) were
also revealed along this profile at the same location in northeast-southwest direction (Figure 12b).
The appropriate drilling locations along this profile can be found from 90 m to 105 m, 205 m to 220 m,
255 m to 270 m, 285 m to 295 m, 310 m to 325 m, 330 m to 350 m, 360 m to 380 m and 405 m to 420 m as
shown in Figure 12b.
The graphical plots of resistivity, SP and IP at 90 m and 180 m depth (Figure 13), and magnetic data (Figure 14d) show clear alterations along the main faults. There is good correlation between ERT, JPM and magnetic data to identify the main faults.
4.5. Analysis of Rock Samples
The X-ray Diffraction Technique (XRD) (D/Max-2400, Rigaku, Tokyo, Japan) was carried out for
the mineral analysis of six rock samples collected from different places in the study area as shown
in Figure 15. Four rock samples (1, 2, 3 and 4) from the weathered rock and two samples (5 and 6)
from the bed rock were collected (Table 2). The XRD test was performed in X-ray Diffraction Analysis
Laboratory of Institute of Geology and Geophysics, Chimes Academy of Sciences. Before the test,
rock samples were grinded in the laboratory with the maximum particle size not more than 0.05 mm.
The mineral analysis was performed using the instrument namely D/Max-2400 XRD ray diffractometer
with the experiment conditions set as 40 kV and 60 mA with Cu target. The interpreted minerals were
characterized as major (>50%), secondary (10–30%) and minor (5–10%). The results summarized in
Table 2 show quartz as the major mineral; microcline, kaolinte, albite and zinnwaldite as the secondary
minerals; and halloysite, perlite sodalite and akerite as the minor minerals in the investigated area.
According to the XRD results, it can be found that samples 1 and 2 contain quartz up to 90% whereas
other four samples contain quartz > 50% suggesting that quartz is the dominant mineral in the study
area. The bedrock in the site area should be medium-acidic rock. The weathered rocks contain a certain
amount of clay minerals such as kaolinite, halloysite, microcline and perlite especially the residual
soil (sample 4) contains 10% to 30% kaolinite. The XRD results show that the residual kaolinite is
mainly due to the weathering and conversion of feldspar, which often occurs in mountain basins and
reflects the warm and humid climate. Fault sample (sample 3) contains pearlite (kaolinite), also known
as pearl clay, which is related to the action of low-temperature hydrothermal fluids and reflects the
tectonic movement of the faults. The bedrock samples contain the minerals such as quartz (>50%),
albite and zinnwaldite (10–30%), and sodalite and akerite (5–10%).
Table 2. The results of XRD for mineral analysis.
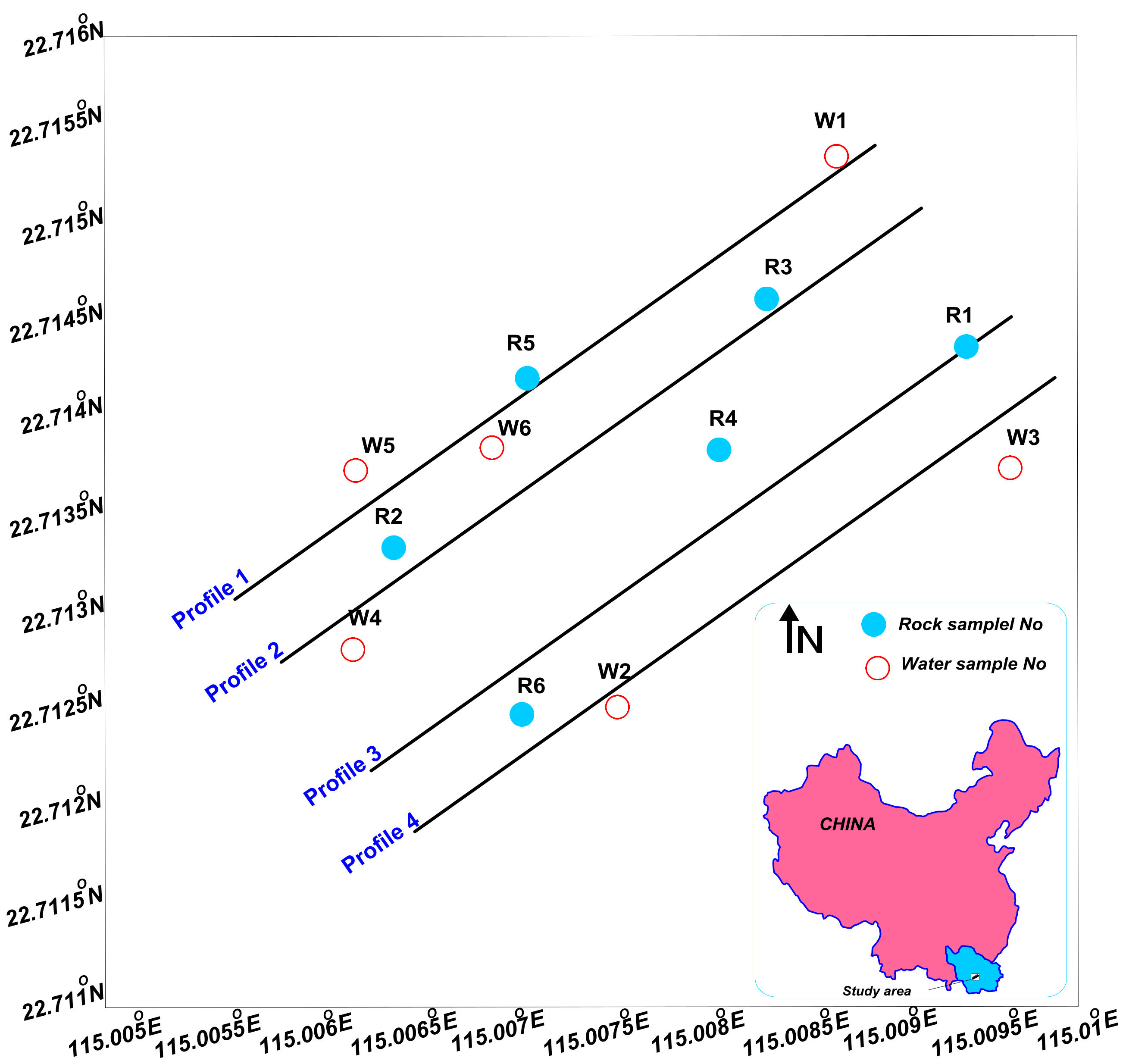
4.6. Analysis of Water Samples
Water samples were collected from six different places in the investigated area as shown in
Figure 15. Two (sample No. 1 and 2) of these six samples were collected from the fissure water (from
bore-wells at an average depth of 40 m to 50 m) and other four samples (sample No. 3, 4, 5 and 6) were
taken from the water channels. Water samples were collected using 500 mL plastic bottles of mineral
water at noon. After the bottles were filled with water, bottle caps were tightened and sealed to avoid
contamination. Before sample collection, the bottles were rinsed three to five times with water filtered
through 0.5 mm mixed cellulose ester membrane. The samples were preserved at approximately
4
◦C before the analysis. The water samples were analyzed as per standard procedure [56–58]
for physicochemical parameters such as sodium (Na+
),potassium (K+
), calcium (Ca2+), magnesium
(Mg2+), bicarbonate (HCO3
−), chloride (Cl−), sulfate (SO4
2−), ammonium (NH4
+
), nitrate (NO3
−), pH,
electrical conductivity (EC) and total dissolved solids (TDS) in the Guangdong Geological Experimental
Testing Center. The international standard referential materials and the synthetic solutions were applied
to the samples in order to verify the accuracy. The physicochemical parameters were calculated using
mean values. For the standard referential materials, recovery was found greater than 95%. To check
the data quality, the charge balance for each water sample was determined and charge balance error
for each analysis was found to be <±5% which is acceptable as shown in Table 3. The major element
compositions of the samples were all lower than the guideline values for drinking water of the World
Health Organization (WHO). However, we are not able to comment on the suitability of the waters for
drinking in the absence of data on trace elements, organic constituents or pathogens. The pH values of
six water samples are more than 7, so all of them are alkaline. However, the pH values of two samples
(fissure water) are above 7.5, which are more alkaline than other four samples. pH values of four
samples (water channels) are low probably due to the faster water channels flow and greater contact
area with air than the fissure water, dissolving more CO2.
Table 3. The results of physicochemical analysis for groundwater samples
5. Discussion
In this investigation, ERT method in combination with JPM, magnetic and borehole data was
used to assess the groundwater potential in the hard rock aquifers of complex geological settings.
Four profiles were interpreted using ERT to map the subsurface weathered/unweathered layers for
groundwater occurrence. Most heterogeneous profiles 1 and 4 were interpreted by the integration of
ERT with JPM and magnetic data for the delineation of main faults in the study area. The interpretation
shows that the study area has complex geological environment with weathered, fractured, fissured,
and faulted zones. The integrated results reveal that the top thick layer is highly weathered overlying
the partly weathered/fissured layer. These two layers (highly weathered and partly weathered) are
highly promising water-bearing strata in the investigated area. Three main faults (F1, F2 and F3)
were delineated along four profiles showing northeast–southwest orientation. The identified faults
show good consistency along all profiles. It was found that highly weathered, partly weathered
and fault zones contain high potential groundwater reserves in the investigated area. A graphical
representation of the measured parameters (topography, water table, weathered-partly weathered
curve, unweathered-partly weathered curve, and main faults) is shown in Figure 16. Water table
was measured two times, i.e., in December 2016 and September 2017. An increment in the water
table was observed in September 2017 during the monsoon rainy season. Figure 17 shows the
subsurface layers (highly weathered, partly weathered, and unweathered layers interpreted by ERT),
the main faults (F1, F2 and F3 revealed by the integration of ERT with JPM and magnetic method),
the topographic variations and the groundwater flow direction. In this study, ERT method was used to
map the subsurface layers (highly weathered, partly weathered, and unweathered layer) in particular.
ERT sections show various discontinuities along different profiles, since ERT provides subsurface
information up to 50 m depth so it is not easy to identify the deep buried faults existing along all
profiles. To identify the main faults extending from profile 1 to profile 4, ERT was integrated with Joint
Profile Method (JPM) and magnetic method. JPM gives joint curves of resistivity, SP, and IP at greater
depth of 90 and 180 m. The alterations in the curves of resistivity, SP, IP and magnetic were carefully
observed and integrated with ERT to delineate the main faults (F1, F2 and F3). The identified faults
extend from profile 1 to profile 4 in northeast-southwest direction, and are confirmed by the different
methods (ERT, JPM and magnetic method). The interpreted faults also show good consistency along
all profiles. The results of XRD technique shows that quartz is the major mineral, whereas microcline,
kaolinite, albite, zinnwaldite are the secondary minerals; and halloysite, kaolinite, perlite, sodalite and
ankerite are the minor minerals. The results of physicochemical analysis reveal that the water samples
lie within the limit suggested by WHO.
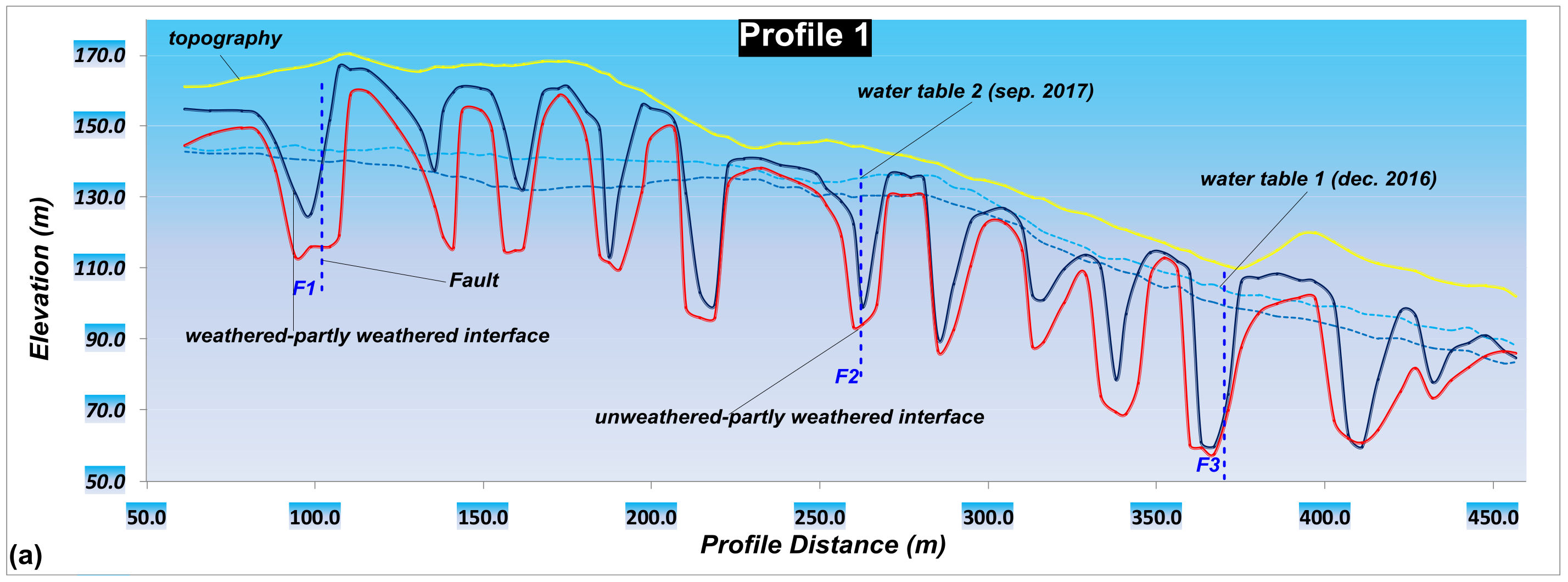
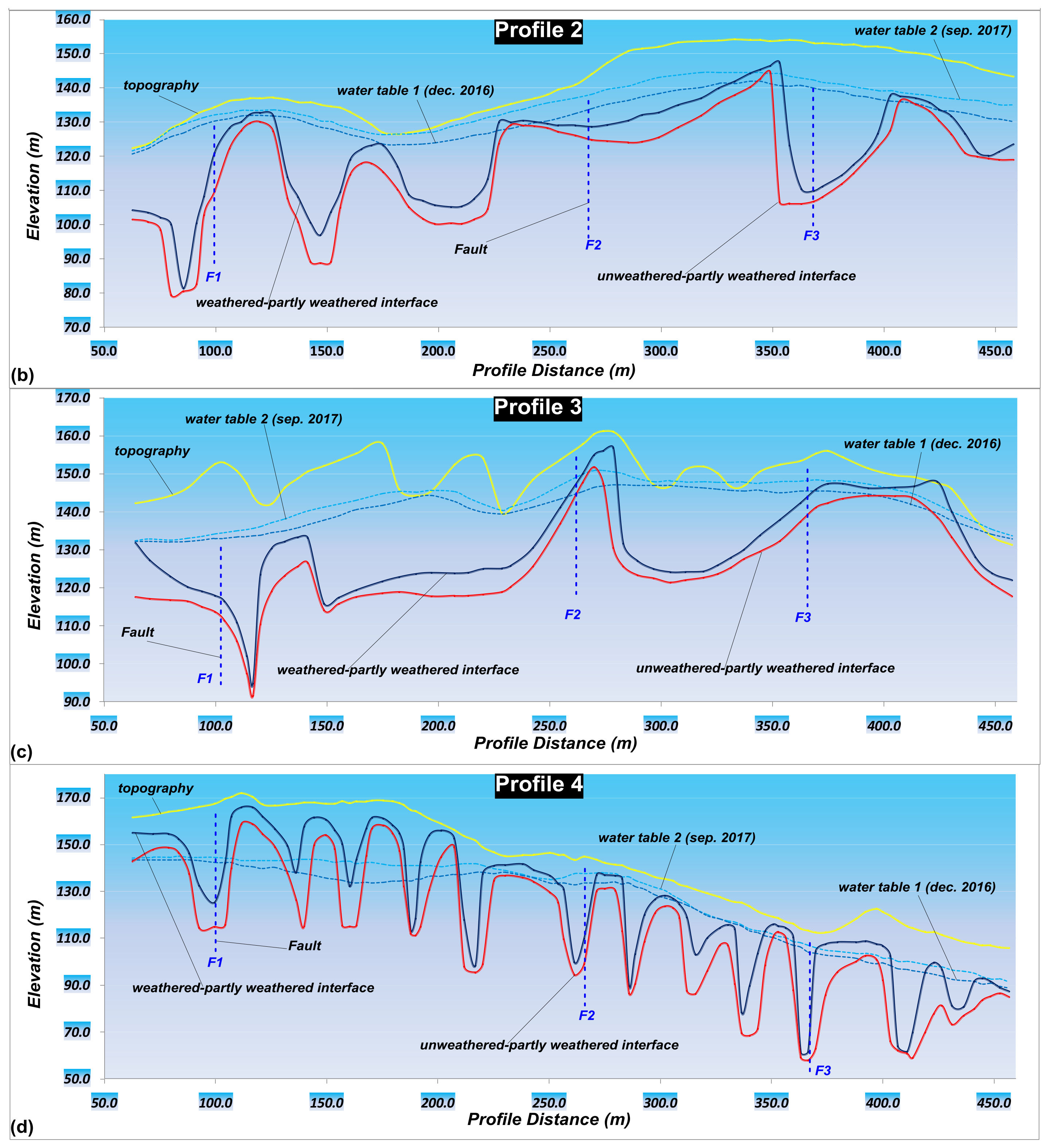
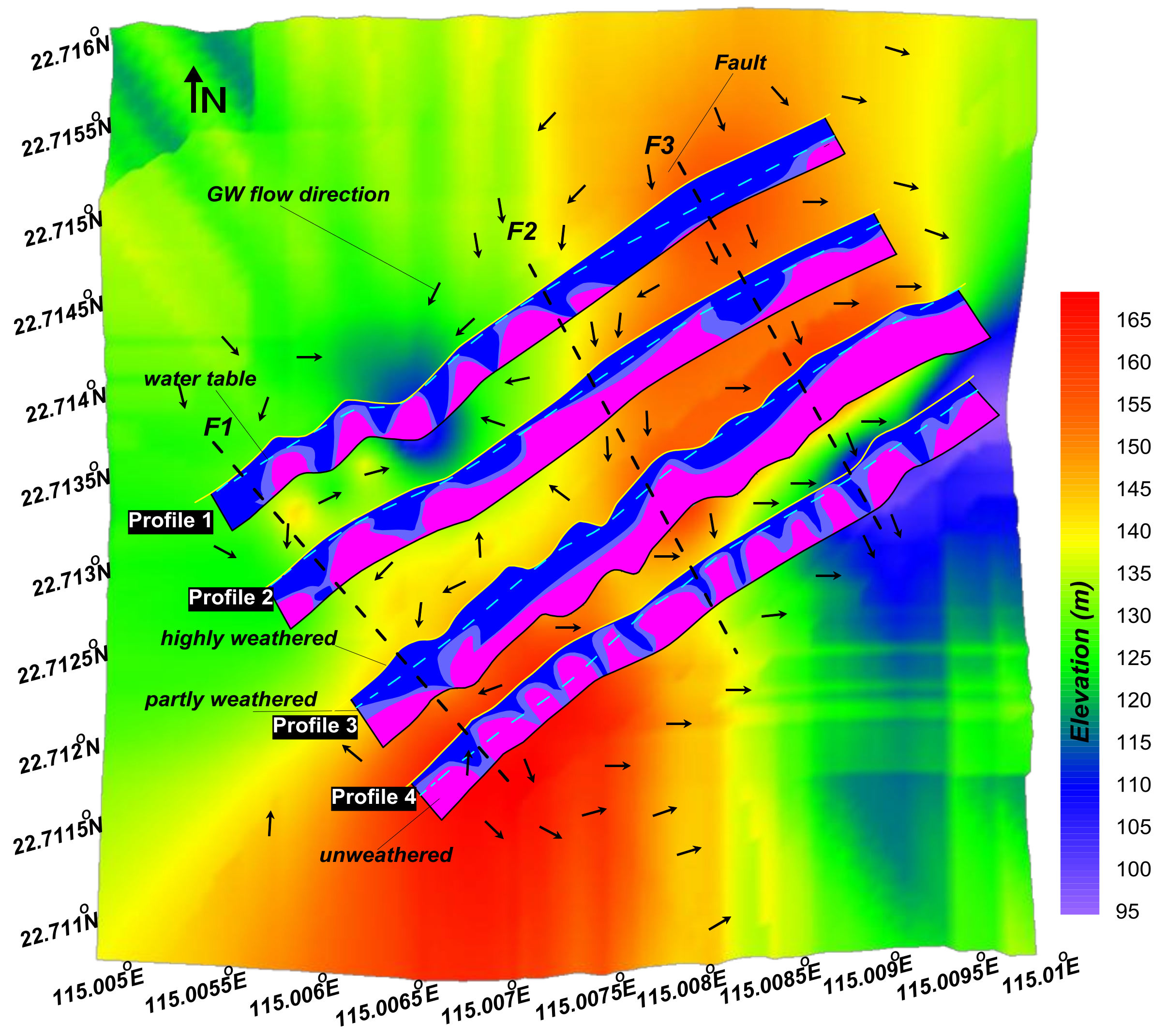
6. Conclusions
In the ERT imaging method, four profiles were performed along the ground surface. The electrical
imaging is more successful technique when it is compared with other geophysical methods to
get a more detailed view of the subsurface features for better understanding of the groundwater
occurrence in the weathered conditions. ERT is an inversion technique which can map the subsurface
conditions even in the complex geological settings. This imaging method was performed using
pole-dipole configuration and RES2DINV software to investigate the groundwater conditions in
weathered environment of complex geology in the study area. The results revealed three distinct
layers along four profiles in the study area based on the correlation of ERT resistivity images with the
borehole data. The first distinct layer underlying the top surface is highly weathered layer mainly
composed of weathered tuff, weathered fissure tuff, silt and clay and other weathered materials with
an average thickness of 20 m and resistivity range of 22Ω m–345 Ωm. The second prominent layer is
partly weathered underneath the top weathered layer with resistivity range of 324 Ωm–926 Ωm and
an average thickness of 10 m mainly consisting of weathered tuff, weathered fissure tuff and other
rocks/minerals such as volcano dust, matrix, feldspar, quartz, pyrite sulfide etc. These two layers
are highly promising water-bearing strata in the study area. Generally, the groundwater reserves
are contained within these two layers. The next layer underlying the partly weathered layer is
unweathered which is fresh basement rock found at an average depth of 20 m with resistivity ranging
from 913 Ωm to 2579 Ωm mainly composed of fresh tuff, fissured tuff with the rocks/minerals such
as volcano dust, matrix, feldspar, quartz, pyrite sulfide etc. The integration of ERT with JPM and
magnetic data reveals three main faults in the study area, which are consistent along all profiles.
The weathered/partly weathered layers with low resistivity values clearly indicate the groundwater
occurrence in the study area because the resistivity of rock decreases with the increasing of percent
water saturation. The results reveal that weathered/partly weathered and faults zones are the best
water-bearing strata for the groundwater potential in the investigated area.